DEFINITION
Electrolysis is simply using electricity to cause the decomposition of water (H2O) into hydrogen gas (H2) and oxygen gas (O2).1 Electrolysis was discovered in 1800 by Anthony Carlisle and William Nicholson2 and is now the primary method of mass-producing hydrogen gas for energy.3
SIMPLE EXPLANATION
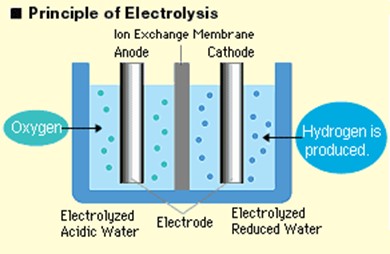
Electrolysis of pure water is very difficult,4 but adding only a small amount of ions makes the process easily achieved. In most places, there are enough minerals in the water that the ionic strength or conductivity of the water is great enough for electrolysis5 to effectively occur without needing to add additional ions to the water (this is why you don’t want to put your toaster or hair drier in water).6
Water dissociates into H+ ions and OH– ions;7 the H+ ions are attracted to the negative electrode (the cathode) and are converted (reduced) to a hydrogen atoms (H) (i.e. e- + H+ => H). This is a highly unstable configuration, and therefore immediately reacts with another hydrogen atom to produce H2, molecular hydrogen gas.8
At the other electrode (the anode), oxidation occurs. The OH– ions are attracted to the positive electrode where they are oxidized to form oxygen gas (O2) and hydrogen ions (H+). However, if chloride is present, it will oxidize (instead of the OH– ions) and form chlorine gas, which will then react with the water to form hypochlorous acid.8
- The cathode reaction is: 2H+ + 2e- (cathode) => H2 (g)
- The anode reaction is: 2OH– => 4e-(anode) +O2 + 2H+
- The overall reaction is: 2H2O => 2H2 (g) + O2 (g)
A MORE COMPLETE EXPLANATION
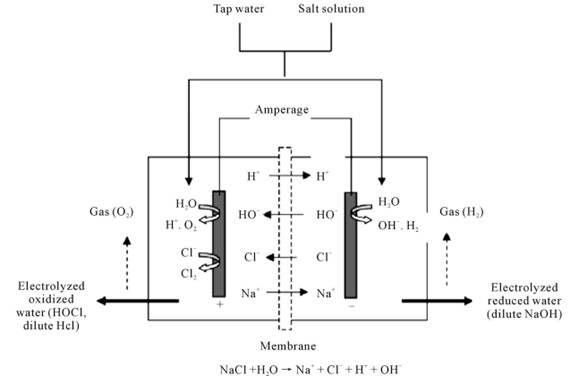
When current is applied to the water, the cations and anions travel to the anode and cathode respectively where they undergo either oxidation or reduction as appropriate.9 The hydronium ions migrate at high mobility via the Grotthuss mechanism (i.e. proton hopping)10 to the cathode where a hydrogen ion (H+) adsorbs to the platinum electrode11 leaving the product of water behind. The proton is reduced to its monoatomic radical species (H) and is now a singly-linked hydrogen atom that diffuses around in two dimensions until it reacts with another radical hydrogen atom to form hydrogen gas.12 The H2 gas may enter nanoscopic cavities in the liquid water13 (nanobubbles),14 or it can exist as a solvated hydrogen molecule14, para-H2 and ortho-H2,15 confined inside the small dodecahedral (H2O)20 cage16 of the sII clathrate hydrate17 (based on the coupled translation-rotation eigenstates).18
Oxidation of anions occurs at the anode.19 Hydroxide ions (OH–) migrate via the transient hyper-coordination of the hydroxides20-21 to the anode where they are oxidized to form molecular oxygen. However, because water is a poor conductor of electricity, usually salts containing chloride (e.g. NaCl, KCl, Ca(Cl)2, etc.) have been added, or are present in tap and ground waters. This produces competing half-reactions at the anode;7 the overpotential for the oxidation of chloride to chlorine is lower than the overpotential for the oxidation of water to produce oxygen. Therefore, chloride preferentially undergoes oxidation at the anode to form chlorine (Cl2) gas, which reacts with water to form hypochlorous acid (H2O + Cl2 => 2HOCl).8
Reactions for electrolysis of water:
- The cathode reaction is: 4H2O (l) + 4e- (from cathode) => 2H2 (g) + 4OH– (aq)
- The anode reaction is: 6H2O (l) => 4e- (to anode) +O2 (g) + 4H3O+ (aq)
- The overall reaction is: 2H2O (l) => 2H2 (g) + O2 (g)
EFFECTS ON PH
At the anode, acid (the hydronium ion (H3O+) is produced; however, at the cathode an equal concentration of base, the hydroxide ion (OH–), is produced. Therefore, if the two compartments are able to mix together, no change in pH of the water will occur. There are small changes in pH near the vicinity of each electrode, but once allowed to mix with the rest of the water, the pH is not altered.
If you place a membrane between the two compartments, which prevents the waters from mixing, then you will produce alkaline water at the cathode and acidic water at the anode. Because pH is a measure of the H+ ion, at the cathode the H+ ions are being converted (reduced) to hydrogen gas. The decrease in the concentration of H+ ions results in a more alkaline pH. Conversely, H+ions are produced at the anode, and so the pH is going to be more acidic. This is how most “water ionizers”/”electrolyzers”) work.
Read More About Understanding pH